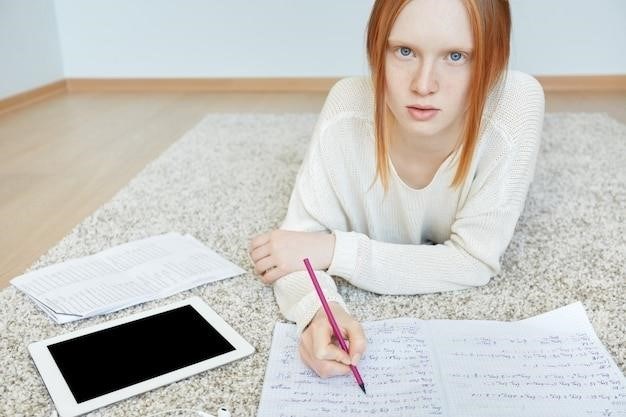
VSEPR Theory and Molecular Geometry
VSEPR theory, or Valence Shell Electron Pair Repulsion theory, is a model used to predict the geometry of molecules based on the repulsion between electron pairs in the valence shell of the central atom․ This theory helps us understand the three-dimensional arrangement of atoms in molecules and predict their shapes and bond angles․
Introduction to VSEPR Theory
VSEPR theory, short for Valence Shell Electron Pair Repulsion theory, is a fundamental concept in chemistry that helps us understand the three-dimensional shapes of molecules․ It’s based on the idea that electron pairs in the valence shell of an atom repel each other, and they arrange themselves in a way that minimizes these repulsions․ This arrangement determines the overall shape of the molecule․
The foundation of VSEPR theory is the understanding that electron pairs, whether they are bonding pairs (shared between two atoms) or lone pairs (not involved in bonding), occupy specific regions of space around the central atom․ These regions of electron density repel each other, and the geometry of the molecule is a consequence of minimizing these repulsions․ The VSEPR model allows us to predict the shape of a molecule based on the number of electron pairs around the central atom, including both bonding and lone pairs․
Understanding VSEPR theory is crucial for predicting molecular geometry, which in turn influences the molecule’s reactivity, physical properties, and even biological functions․ By applying the VSEPR model, we can visualize the arrangement of atoms in space and gain insights into the behavior of molecules in various chemical and biological processes․
Key Terms and Concepts
To effectively predict and understand molecular geometries using VSEPR theory, it’s essential to grasp a few key terms and concepts⁚
- Electron Domain⁚ This refers to a region around the central atom where electrons are concentrated․ It can be a single bond, a double bond, a triple bond, or a lone pair of electrons․
- Electron Pair Geometry⁚ This describes the arrangement of electron domains around the central atom․ Common electron pair geometries include linear, trigonal planar, tetrahedral, trigonal bipyramidal, and octahedral․
- Molecular Geometry⁚ This refers to the three-dimensional arrangement of atoms in a molecule, considering only the positions of the atoms, not the lone pairs․ The molecular geometry can be the same as the electron pair geometry or different, depending on the presence and arrangement of lone pairs․
- Bond Angle⁚ This is the angle formed between two adjacent bonds in a molecule․ Bond angles are influenced by the repulsion between electron domains and can vary depending on the molecular geometry․
- Lone Pair⁚ A pair of electrons that are not involved in bonding․ Lone pairs have a stronger repulsive effect than bonding pairs, leading to distortions in bond angles and molecular shapes․
- Hybridization⁚ This is the mixing of atomic orbitals to form new hybrid orbitals that have different shapes and energies․ Hybridization is often used to explain the bonding and geometry in molecules․
Understanding these concepts is fundamental to successfully applying VSEPR theory to predict and interpret molecular shapes․
Steps for Predicting Molecular Geometry
Predicting molecular geometry using VSEPR theory involves a systematic approach that can be broken down into a series of steps⁚
- Draw the Lewis Structure⁚ Start by drawing the Lewis structure of the molecule or ion․ This will show you the arrangement of atoms and the bonding and non-bonding electrons․
- Count the Electron Domains⁚ Determine the number of electron domains around the central atom․ Remember that each single, double, or triple bond counts as one electron domain, and lone pairs also count as one electron domain․
- Determine the Electron Pair Geometry⁚ Based on the number of electron domains, identify the electron pair geometry using the following table⁚
- 2 electron domains⁚ Linear
- 3 electron domains⁚ Trigonal planar
- 4 electron domains⁚ Tetrahedral
- 5 electron domains⁚ Trigonal bipyramidal
- 6 electron domains⁚ Octahedral
- Determine the Molecular Geometry⁚ Consider the presence and arrangement of lone pairs․ Lone pairs have a stronger repulsive effect than bonding pairs, so they can distort the molecular geometry․ Use the table above and adjust for lone pairs to find the molecular geometry․
- Predict Bond Angles⁚ Estimate the bond angles based on the molecular geometry; Ideal bond angles for common geometries are as follows⁚
- Linear⁚ 180°
- Trigonal planar⁚ 120°
- Tetrahedral⁚ 109․5°
- Trigonal bipyramidal⁚ 90°, 120°
- Octahedral⁚ 90°
Note that lone pairs can cause deviations from these ideal bond angles․
By following these steps, you can systematically predict the molecular geometry of a molecule or ion using VSEPR theory․
VSEPR Practice Problems with Answers
This section provides a collection of practice problems to help you solidify your understanding of VSEPR theory and molecular geometry prediction․ Each problem is accompanied by a detailed solution, allowing you to check your work and learn from any mistakes․
Problem 1⁚ Predicting Molecular Geometry
Predict the molecular geometry of the following molecules using VSEPR theory⁚
- a) H2S
- b) CF4
- c) HCN
- d) NF3
Answers⁚
- a) H2S⁚ The central sulfur atom has two bonding pairs and two lone pairs․ This leads to a bent or V-shaped molecular geometry with an approximate bond angle of 104․5 degrees․
- b) CF4⁚ The central carbon atom has four bonding pairs and no lone pairs․ This results in a tetrahedral molecular geometry with ideal bond angles of 109․5 degrees․
- c) HCN⁚ The central carbon atom has one bonding pair and two lone pairs, giving a linear molecular geometry with a bond angle of 180 degrees․
- d) NF3⁚ The central nitrogen atom has three bonding pairs and one lone pair, leading to a trigonal pyramidal molecular geometry with an approximate bond angle of 107 degrees․
Problem 2⁚ Determining Bond Angles
Determine the approximate bond angles in the following molecules or ions using VSEPR theory⁚
- a) CO32-
- b) NO3-
- c) NO2
- d) NO2-
Answers⁚
- a) CO32-⁚ The central carbon atom has three bonding pairs and no lone pairs․ This results in a trigonal planar molecular geometry with ideal bond angles of 120 degrees․
- b) NO3-⁚ Similar to CO32-, the central nitrogen atom also has three bonding pairs and no lone pairs, resulting in a trigonal planar molecular geometry with ideal bond angles of 120 degrees․
- c) NO2⁚ The central nitrogen atom has two bonding pairs and one lone pair․ This leads to a bent molecular geometry with a bond angle slightly less than 120 degrees due to the repulsion from the lone pair․
- d) NO2-⁚ Similar to NO2, the central nitrogen atom has two bonding pairs and one lone pair, resulting in a bent molecular geometry with a bond angle slightly less than 120 degrees due to the repulsion from the lone pair․
Problem 3⁚ Identifying Lone Pairs and Bonding Pairs
For each of the following molecules, identify the number of lone pairs and bonding pairs on the central atom⁚
- a) H2S
- b) CF4
- c) HCN
- d) NF3
- e) BCl3
- f) NH2Cl
- g) OF2
- h) H3O+
- i) PCl3
- j) ClO3-
Answers⁚
- a) H2S⁚ 2 lone pairs, 2 bonding pairs
- b) CF4⁚ 0 lone pairs, 4 bonding pairs
- c) HCN⁚ 0 lone pairs, 2 bonding pairs
- d) NF3⁚ 1 lone pair, 3 bonding pairs
- e) BCl3⁚ 0 lone pairs, 3 bonding pairs
- f) NH2Cl⁚ 1 lone pair, 3 bonding pairs
- g) OF2⁚ 2 lone pairs, 2 bonding pairs
- h) H3O+⁚ 1 lone pair, 3 bonding pairs
- i) PCl3⁚ 1 lone pair, 3 bonding pairs
- j) ClO3-⁚ 1 lone pair, 3 bonding pairs
VSEPR Theory Applications
VSEPR theory has numerous applications in various fields, including predicting molecular polarity, understanding hybridization, and explaining chemical and biological reactions․
Predicting Molecular Polarity
VSEPR theory plays a crucial role in determining the polarity of a molecule․ Molecular polarity arises from the uneven distribution of electron density within a molecule, leading to a separation of charge․ A molecule is considered polar if it possesses a net dipole moment, which is a measure of the overall polarity of the molecule․ This dipole moment results from the vector sum of individual bond dipoles, which are created by the difference in electronegativity between the bonded atoms․
VSEPR theory helps predict the molecular geometry, which directly influences the arrangement of bond dipoles․ For instance, a molecule with a symmetrical geometry, such as tetrahedral or linear, may have individual bond dipoles that cancel each other out, resulting in a non-polar molecule․ In contrast, a molecule with an asymmetrical geometry, such as bent or trigonal pyramidal, may have bond dipoles that do not cancel, leading to a net dipole moment and a polar molecule․
By understanding the relationship between molecular geometry and polarity, VSEPR theory allows us to predict the polarity of a molecule based on its Lewis structure and predicted shape․ This knowledge is essential for understanding various chemical and physical properties, such as solubility, boiling point, and intermolecular interactions․
Understanding Hybridization
Hybridization is a theoretical concept in chemistry that explains the bonding and geometry of molecules․ It involves the mixing of atomic orbitals to form new hybrid orbitals that are suitable for bonding․ The process of hybridization is driven by the desire of atoms to achieve a more stable electronic configuration, which is often achieved by maximizing the overlap of orbitals․
VSEPR theory helps us understand the hybridization of central atoms in molecules․ The number of electron pairs around the central atom, including both bonding and lone pairs, determines the hybridization scheme․ For instance, a central atom with four electron pairs will undergo sp3 hybridization, resulting in four equivalent sp3 hybrid orbitals that are directed towards the corners of a tetrahedron․ Other common hybridization schemes include sp2 for three electron pairs (trigonal planar geometry) and sp for two electron pairs (linear geometry)․
Hybridization is a powerful tool for predicting the bonding and geometry of molecules․ It helps explain the formation of sigma and pi bonds, the strength of bonds, and the overall shape of molecules․ Understanding hybridization is essential for comprehending the structure and reactivity of molecules in chemistry․
Applications in Chemistry and Biology
VSEPR theory has wide-ranging applications in various fields of chemistry and biology, providing a fundamental framework for understanding the structure and reactivity of molecules․ In organic chemistry, VSEPR theory helps predict the shapes of complex molecules, guiding the understanding of their reactions and properties․ For instance, it helps explain the geometry of alkanes, alkenes, and alkynes, crucial for understanding their reactivity and physical properties․ In inorganic chemistry, VSEPR theory is essential for understanding the structure and bonding of coordination complexes, which play a vital role in catalysis, materials science, and biological systems․
VSEPR theory also extends to biological systems, where its applications are crucial for understanding the structure and function of biomolecules․ It helps predict the shapes of proteins, nucleic acids, and enzymes, which are fundamental building blocks of life․ Understanding the shapes of these biomolecules is essential for comprehending their interactions, catalytic activities, and overall biological functions․ VSEPR theory also plays a crucial role in drug design, where understanding the shapes of molecules helps design drugs that bind to specific target molecules in the body․
The applications of VSEPR theory are vast and continue to be explored in various fields․ Its ability to predict molecular geometry and explain the structure and reactivity of molecules makes it an indispensable tool in modern chemistry and biology․
Resources and Additional Information
For further exploration and practice, numerous resources are available online and in textbooks, offering a wealth of information and exercises․
VSEPR Theory Worksheets and Practice Problems
Numerous websites and educational resources offer VSEPR theory worksheets and practice problems to enhance understanding and proficiency in predicting molecular geometry․ These worksheets typically provide a series of molecules or ions, requiring students to draw Lewis structures, determine the number of electron domains around the central atom, and predict the electron domain and molecular geometries using VSEPR theory․ The worksheets may also include questions about bond angles, hybridization, and molecular polarity․
Some websites even provide answer keys to help students check their work and identify areas where they might need further clarification․ These practice problems are invaluable for solidifying understanding of VSEPR theory and its application in predicting molecular shapes․
Online VSEPR Simulation Tools
The advent of online VSEPR simulation tools has revolutionized the way students and educators learn and explore molecular geometry․ These interactive tools allow users to build molecules virtually, manipulate their structure, and visualize the effects of electron pair repulsion on molecular shape․
Many online VSEPR simulation tools provide a user-friendly interface, allowing users to select different atoms, adjust bond lengths and angles, and observe the resulting molecular geometry in 3D․ Some tools also include features for calculating bond angles, determining molecular polarity, and visualizing hybridization․ These simulations offer a dynamic and engaging way to understand VSEPR theory and its implications for molecular structure․
VSEPR Theory in Textbooks and Articles
VSEPR theory is a fundamental concept in chemistry, and its application to predict molecular geometry is widely discussed in textbooks and articles; General chemistry textbooks typically devote a chapter or section to VSEPR theory, explaining its principles, providing examples of molecular shapes, and offering practice problems for students to test their understanding․
Specialized textbooks on inorganic chemistry, physical chemistry, and molecular modeling also cover VSEPR theory in greater detail, exploring its theoretical basis, limitations, and applications in different areas of chemistry․ Numerous scientific articles and research papers delve into the theoretical aspects of VSEPR theory, its refinements, and its role in understanding chemical bonding and molecular properties․